Fluorescent technologies for intraoperative parathyroid identification
Introduction
Accurate and expedient identification of parathyroid glands is crucial to successful thyroid and parathyroid surgery. Inadvertent injury or resection of the parathyroids during total thyroidectomy leads to hypoparathyroidism, a common complication associated with long-term morbidity (1,2). During parathyroid surgery, inability to detect a hyperfunctioning parathyroid adenoma results in failed parathyroidectomy, and inability to localize and confirm normal glands may result in lengthy and unnecessary additional exploration. Typically, parathyroid glands are identified intraoperatively by visual inspection. Needle aspiration for parathyroid hormone (PTH) and frozen section pathology can be useful intra-operative adjuncts, but are only suitable for confirmation of identified glands; neither method can help surgeons localize parathyroid glands or ensure an intact blood supply. Moreover, both methods require time and additional cost for analysis outside of the operating room.
In order to achieve real-time intraoperative identification and confirmation of parathyroid glands without physically excising tissue—some call it an “optical biopsy”—several fluorescence techniques have been investigated. Fluorescence occurs when a substance absorbs radiation (in the form of light or X-rays) at one wavelength, and quickly emits radiation at a longer wavelength. Chemical compounds with fluorescent properties, called “fluorophores”, can be naturally occurring in some tissues, causing “autofluorescence” when excited by an external light source. Alternatively, extrinsic fluorophores, such as contrast agents, can be added to enhance tissue fluorescence. To optimally produce and detect fluorescence, systems and devices may generate and filter particular wavelengths for excitation, detect and filter fluorophore emissions, and process and relay emission data to the user.
Intrinsic near-infrared autofluorescence (NIRAF) of parathyroid glands was first described in 2011 by researchers at Vanderbilt University, who noted that parathyroid fluorescence was consistently higher than that of the thyroid and other surrounding tissues, both in normal and hyperfunctioning glands (3). Near-infrared (NIR) light is particularly useful for intra-operative imaging guidance because it is safe, invisible (causing no disruption to visualization of the operative field), and because its longer wavelengths allow deeper penetration into living tissue (4,5). Currently, two devices are approved by the U.S. Food and Drug Administration (FDA) that use NIRAF-based real-time detection of parathyroid tissue: Fluobeam® (Fluoptics, Grenoble, France) and the Parathyroid Detection PTeye® System. (AiBiomed Corp., Carlsbad, CA, USA) (6).
Autofluorescence, because it is based on intrinsic properties of the parathyroid tissue, does not depend on, nor can it evaluate, the viability of tissue (7). However, when NIR fluorescent dye indocyanine green (ICG) is injected intravascularly, the additional fluorescent contrast allows for real-time assessment of vascularization and perfusion of parathyroid tissue (8). In this article, we will provide a brief description of fluorescent technologies used for the identification of parathyroid glands, and describe their applications for thyroid and parathyroid surgery.
Parathyroid fluorescence in thyroid surgery
Hypoparathyroidism is the most common complication of total thyroidectomy and re-operative thyroid surgery, with a median incidence of temporary hypoparathyroidism of approximately 27% (9,10). Although transient hypoparathyroidism is rarely a difficult clinical problem because it is effectively treated with oral calcium, permanent hypoparathyroidism requires lifelong therapy and lead to significant morbidity, as well as increased mortality (2,11). Impaired parathyroid function after thyroid surgery can be caused by intentional or unintentional removal of parathyroid glands, as well as thermal or mechanical injury, or disruption of the glands’ delicate arterial supply or venous drainage.
Surgeons aim to preserve all four parathyroid glands during thyroid surgery, but resection of one or more glands may be necessary based on the extent of thyroid disease. In order to safely preserve as many glands as possible, surgeons must be able to recognize and identify parathyroid tissue, which can be challenging due to their small size and similar color to the surrounding thyroid, lymph nodes, and fatty tissue. Experienced surgeons use anatomical landmarks to mentally map expected locations and attentively explore those areas for visual confirmation (9). Once the glands are identified visually, if necessary, parathyroid tissue can be confirmed with either needle aspiration for PTH or frozen section pathologic examination. However, despite these measures, incidental parathyroidectomy is common, and has been noted in approximately 16–22% of thyroid surgeries (12-15). Parathyroid glands that have been devascularized in the course of dissection can be autotransplanted into intramuscular pockets with good success (16). End of case or immediate postoperative measurement of serum PTH is highly accurate for predicting symptomatic postoperative hypocalcemia (17), but is not a preventative measure.
NIRAF in thyroid surgery
Fluorescence with NIR light can be used to help identify and preserve parathyroid glands during dissection, either through detection of autofluorescence from the glands themselves, or by contrast-enhanced fluorescence using injectable fluorescent dye. The responsible fluorophore for NIRAF within the parathyroid glands remains unknown. However, several theories have been suggested, including those attributing autofluorescence to calcium and vitamin D receptors, a difference in cellular density, and the presence of a pseudo-colloid in the glands (18). NIRAF persists after parathyroid resection, and remains even after formalin fixation or cryopreservation, indicating that the responsible fluorophore is a chemical structure inherent to the gland, and not dependent upon perfusion or gland viability (7,19,20). In this section, we will discuss the two FDA approved systems (image-based and probe-based) and discuss their unique strengths and weaknesses.
Image-based NIRAF systems
The Fluobeam® Systems (both the original Fluobeam® 800 Clinical System and the updated Fluobeam® LX) (Figure 1) excite tissues at 750 nm. The device then collects signals for wavelengths over 800 nm and displays them as video images in real time (7). The probe is covered with a sterile sleeve for intra-operative use, but no part of the system directly touches the patient. The working distance of the probe is approximately 20 cm. Video or still images are stored and may be analyzed post hoc for quantification of fluorescence.
The reported sensitivity of the Fluobeam® device ranges from 94–100% (7,21-23). Several studies of patients undergoing total thyroidectomy with the Fluobeam® device have demonstrated a higher parathyroid identification rate and lower rate of postoperative hypocalcemia (24,25). In an international multicenter trial, nearly half (46%) of parathyroid glands were identified with NIRAF first before being identified with the naked eye. Notably, smaller parathyroid glands were less likely to be localized with visual exploration than with NIRAF (21).
In a multicenter randomized trial by Benmiloud et al., NIRAF was found to reduce the risk of post-operative hypocalcemia in a multivariate analysis adjusting for center and surgeon heterogeneity and confounders. In this study, fewer patients underwent parathyroid autotransplantation in the NIRAF group, and the number of inadvertently resected parathyroid glands was also significantly lower (26). Other studies, however, have found no difference in postoperative hypocalcemia between NIRAF and control groups, nor in rates of parathyroid autotransplantation (27,28), and results on inadvertent parathyroidectomy are mixed.
Probe-based NIRAF systems
The PTeye® system includes a disposable, sterile, fiber-optic probe, connected to a console containing a NIR diode laser, detector, and interactive display (Figure 2). The probe serves as a conduit for both the laser and the detector. The tip of the probe touches the tissue to be examined and the laser is activated by a foot pedal. The quantitative fluorescence is displayed, as well as the ratio between the tested tissue and background baseline, and it beeps (auditory signal) if the signal detected is 120% or more of the background. The background is set at start of the case by averaging 5 measurements on different areas of the thyroid gland, and adjusted if necessary by testing it on other parts of the thyroid gland. Because the device tip touches the tissue of interest (thus with minimal distance), the system is not affected by operating room lights or surgical headlamps (29,30).
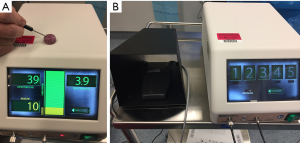
The reported sensitivity of the probe-based PTeye® device and its precursors ranges from 96–100% (5,23,29,31). The pre-commercial system showed peak parathyroid fluorescence to be 1.2 to 29 times than that of thyroid fluorescence, with an overall accuracy of 97% (32). In a study of the commercial PTeye® device, Thomas et al. demonstrated an overall accuracy of 96.1%, which increased to 97.4% when excluding secondary renal hyperparathyroidism, which has been shown to have lower NIRAF intensity, leading to false negative results (29). One study found that pre-operative calcium and vitamin D levels, patient body mass index (BMI), and disease state (benign vs. malignant thyroid disease, hyperthyroidism, or hyperparathyroidism) affect parathyroid fluorescence, while patient age, gender, ethnicity, and PTH levels had no effect (32).
Image based vs. probe-based NIRAF systems
Both image-based and probe-based systems appear to accurately identify parathyroid glands, noninvasively and in real-time. Tips for practical use of these devices during thyroid surgery are discussed in Table 1. Whether they improve clinical outcomes, such as inadvertent parathyroidectomy and post-operative hypocalcemia, is still being investigated. Though both systems are FDA-approved, each has specific benefits and drawbacks. The Fluobeam® systems are contactless, and display a large field of view of all fluorescing tissue. This can be useful for mapping locations of glands, even if they have yet to be visually identified by the surgeon under white light (Figure 3). However, the device is operator-dependent, both in requiring the surgeon to estimate the suggested 20-cm distance from the surgical field and hold the hand-held device steady for optimal imaging, as well as requiring the surgeon to visually interpret the images. Notably, false positive signals have been described with image-based systems both from colloid thyroid nodules and brown adipose tissue (7). Anecdotally, such false positive signals have also been seen with the probe-based system. Although room lights can remain on, the visual signals are better without direct illumination of the field by the spotlights or surgeon’s headlamp, thus requiring the operative lights to be turned off and back on during the procedure. The imaging process takes approximately 1 minute for each area imaged (21).
Table 1
Surgery | Probe-based | Image-based |
---|---|---|
Thyroidectomy (for identification of normal parathyroid glands) | During dissection: candidate parathyroid gland/s can be probed. If high signal and likely to be parathyroid gland/s, surgeon can attempt to protect and keep vascular supply intact | During dissection: image the area exposed before extensive dissection to identify candidate parathyroid gland/s and attempt to protect and keep their vascular supply intact during dissection |
After excision: probe and confirm the parathyroid glands remain in the dissection bed and visually check for likelihood of viability if left in situ | After excision: scan the bed of dissection to identify remaining parathyroid glands and visually check for likelihood of viability if left in situ | |
After excision: visually inspect the thyroid specimen and probe area/s that appears to be parathyroid tissue. If high signal, excise and confirm by PTH aspirate or frozen section, then autotransplant to cervical muscle | After excision: scan the thyroid specimen and identify high signal area/s that may be parathyroid tissue. Excise such tissue and confirm by PTH aspirate or frozen section, then autotransplant to cervical muscle | |
Parathyroidectomy (for identification of both normal and abnormal parathyroid glands) | During exploration: check candidate adenoma/s. High signal can be from either normal parathyroid gland or parathyroid tumor and will require further dissection to confirm | During exploration: scan dissected area. Bright image signal can be from a normal parathyroid gland or a parathyroid tumor and will require further dissection to confirm |
After excision: probe the excised tissue to confirm it has high signal (likely parathyroid). If there is a normal cap on the tumor, expect the normal parathyroid cap to have higher signal than the tumor | After excision: scan the excised tissue to confirm it is bright (likely parathyroid). If there is a normal cap on the tumor, the normal parathyroid cap is usually brighter than the tumor. Large parathyroid tumors can appear heterogenous in brightness | |
After excision: probe the area of excision for potential residual tumor. This should be done especially if a parathyroid tumor was very adherent to the thyroid or if there is concern for a dumbbell shaped tumor that was only partially excised | After excision: scan the area of excision for potential residual tumor. This should be done especially if a parathyroid tumor was very adherent to the thyroid or if there is concern for a dumbbell shaped tumor that was only partially excised |
PTH, parathyroid hormone.
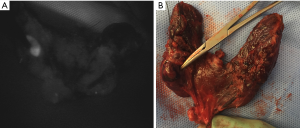
The probe-based PTeye® device is similar in interface and method of use to the nerve monitor probe, already a commonly used device for many thyroid and parathyroid surgeons. However, it requires direct contact with the specific tissue of interest, so a likely parathyroid gland must be identified before “confirmation” with the device. The device also requires an initial calibration to set the background and adds time to the beginning of each procedure.
Notably, both devices are limited by the depth of penetration of light in biological tissue. To be detected, the suspected parathyroid gland must be no deeper than a few millimeters below the overlying tissue (30). Because of this, NIRAF would not be likely to help identify ectopic glands, such as those in the thyroid, mediastinum, or carotid sheath. Of course, of these, only intra-thyroidal glands are at risk of inadvertent removal during thyroidectomy.
Contrast-enhanced parathyroid fluorescence in thyroid surgery
NIRAF is inherent to the chemical makeup of parathyroid gland tissue and cannot be used to evaluate viability or perfusion. However, perfusion to the gland can also be assessed with NIR light by using contrast-enhanced fluorescent dye. The most commonly used dye is ICG, which provides a higher signal-to-background ratio than other agents, with an emission peak at 830–835 nm (30). ICG is a fluorescent dye originally developed for use in NIR photography by Kodak Research Laboratories in 1955 and approved for clinical use in 1956. When injected intravenously, ICG binds to plasma proteins, confining the dye intravascularly. It has a mean half-life of 3.4 minutes and is rapidly taken up by hepatocytes and excreted into bile. These properties have made ICG clinically useful, particularly for angiography (33,34) and biliary imaging (35). ICG does not target parathyroid tissue, but because parathyroid glands are well-vascularized, the intravascular dye highlights the contrast between the gland and the poorly vascularized surrounding tissue, such as fat and thymus. The addition of ICG in the evaluation of parathyroid glands provides increased fluorescence (a brighter image), and enables deeper tissue imaging than NIRAF alone (30).
ICG preparation is similar to that used during abdominal surgery, with 25 mg of powder suspended in 10 mL of sterile water, and 1–3.5 mL of this solution injected through a peripheral vein. Injections can be repeated up to a maximum dose of 5 mg per kg per day (34,36). Approximately 1–2 minutes after injection, a NIR fluorescence imaging camera (e.g., the SPY® system by Stryker; Kalamazoo, MI, USA) is used to acquire images. Imaging systems typically include both a NIR-only view in grayscale, as well as a combined white light and fluorescence overlay view. The newer Fluobeam® LX also has a setting for contrast-enhanced imaging. Similar to image-based NIRAF systems, there is no real-time quantification of fluorescence with ICG contrast-based fluorescence imaging. However, surgeons have used visual scores to assess gland perfusion, with grade 0 = not vascularized (black), grade 1 = moderately vascularized (gray), and grade 2 = well-vascularized (white) (34,37,38). ICG can be administered and fluorescence imaging performed both early in the thyroidectomy dissection to identify parathyroid glands, and at completion of the dissection to assess perfusion and thus viability of the parathyroid glands left in situ. Table 2 provides a practical guide for the use of contrast-enhanced parathyroid imaging during thyroidectomy.
Table 2
Surgery | Use |
---|---|
Thyroidectomy (for identification of normal parathyroid glands and confirm their perfusion) | During dissection: inject contrast when candidate upper and lower parathyroid glands on the side of interest have been found, but just before extensive dissection. Identify parathyroid glands, which will have much higher perfusion. Also note the arterial supply of the parathyroids, especially if it is circuitous, and protect it during dissection |
After excision: inject contrast and inspect the parathyroid glands remaining in the dissected area. Note and grade the perfusion (2 = excellent, 1 = some perfusion, 0 = no perfusion). Non-perfused parathyroid gland can be excised, confirmed by PTH aspirate or frozen section, then autotransplanted to cervical muscle | |
After excision: presence of at least one perfused parathyroid gland on the initial side of dissection is associated with a lower risk of postoperative hypoparathyroidism and may influence the decision to continue dissection on the contralateral side | |
Parathyroidectomy (for identification of both normal and abnormal parathyroid glands and confirm their perfusion) | During exploration: inject contrast when candidate parathyroid tumor is found. Confirm high perfusion and note the vascular supply to aid dissection |
Planning of subtotal parathyroidectomy: expose and dissect all four glands. Inject dye to confirm glands have good perfusion. Partially excise the gland planed for subtotal resection. If the subtotally resected remnant has good perfusion, excise the other glands. If not, subtotally resect the next candidate gland and inject dye to confirm perfusion. This can be repeated until a well perfused remnant is confirmed |
Note: once contrast has been used, the tissue fluorescence signal will overwhelm the ability to detect autofluorescence by either image-based or probe-based device. This needs to be considered in the planning of operation.
The term “ICG angiography” is frequently used synonymously for “ICG fluorescence imaging”. To avoid confusion, we use ICG angiography to mean using ICG to map the vascular supply (definition of “angiography”) and ICG fluorescence imaging to denote both angiography and assessment of perfusion.
In a Swiss study of 36 thyroidectomy patients with at least one well-vascularized parathyroid gland visualized by ICG fluorescence imaging, 100% had normal post-operative PTH levels (34). This finding was confirmed in larger group of 146 patients with at least one well-vascularized gland, who were randomized to receive either standard follow-up with routine calcium supplementation or no calcium supplementation. None of the patients had hypocalcemia or hypoparathyroidism on post-operative day 10 (37). Most studies to date have demonstrated correlation between ICG uptake in the parathyroid glands and post-operative PTH levels (8,39). However, limited results from U.S. studies have been somewhat less definitive. A U.S. study of 86 patients undergoing ICG fluorescence imaging during thyroidectomy found that the sensitivity of at least one well perfused parathyroid gland in predicting normal post-operative PTH was only 58%; two or more well perfused glands increased the sensitivity, but only to 72% (40). An additional U.S. study found no significant difference in post-operative PTH change or post-operative hypocalcemia between groups with good vs. poor ICG perfusion patterns (38). Correlation between parathyroid perfusion on ICG fluorescence imaging and post-operative parathyroid function, as measured by PTH levels, is complicated by the fact that not all parathyroid glands are routinely identified during thyroidectomy. Mechanisms to quantify and/or standardize the evaluation of perfusion with ICG fluorescence may also further clarify the association between ICG fluorescence imaging and parathyroid function, and will provide more robust data for future studies.
The main benefit of ICG over NIRAF is the ability to assess gland perfusion. ICG allows for real-time intraoperative imaging without exposure to radiation. ICG is nontoxic and nonionizing, though the injection solution does contain some sodium iodide, and an allergic reaction is possible, leading many practitioners to avoid use in patients with iodine allergy (33,41). While more data is required to confirm the correlation between ICG fluorescence imaging and post-operative parathyroid function, most studies have found that patients with evidence of good perfusion to at least one to two glands do not develop permanent hypoparathyroidism. While NIRAF can help localize and identify the presence of parathyroid tissue, a devascularized gland has the same appearance as a normal gland, though it will not function postoperatively. Compared to intraoperative serum PTH measurement, another test of postoperative parathyroid gland function that provides a collective assessment of all viable glands, ICG can provide data about individual gland perfusion.
Because of their complementary functions, both NIRAF and ICG contrast-based fluorescence imaging may be used during thyroidectomy to both identify and confirm parathyroid tissue, as well as assess perfusion. However, because of the much stronger fluorescence of ICG, which persists over time, it is not possible to detect the innate autofluorescence of the parathyroid glands even after a single ICG injection. If NIRAF assessment is planned, it should be performed before ICG fluorescence imaging. The residual fluorescence after ICG administration lessens contrast between the parathyroid glands and the surrounding tissue, so while multiple ICG injections are possible, the diminishing contrast should be considered during visual assessment.
Fluorescence in parathyroid surgery
The hallmark of successful parathyroidectomy is the removal of abnormal parathyroid tissue and preservation of normal parathyroid tissue. In 80–85% of cases, primary hyperparathyroidism (PHPT) is caused by a solitary parathyroid adenoma, while four-gland hyperplasia accounts for 10–15% of cases, multiple adenomas for 5%, and parathyroid carcinoma for <1% (42). Pre-operative imaging is often performed to assist the surgeon in localizing the abnormal gland(s) during surgical planning, and can help surgeons select appropriate patients for focused versus bilateral exploration. Although cure rates of parathyroidectomy for PHPT are >95%, intraoperative identification of parathyroid glands can be challenging even for experienced surgeons. Failed explorations and persistent disease result from the inability to detect and remove hyperfunctioning parathyroid glands. Adjuncts such as radioguidance with sestamibi or methylene blue dye injection have been described for intraoperative localization, but have not gained widespread use (41).
Imaged-based and probe-based detection of autofluorescence and ICG fluorescence imaging may be used during parathyroidectomy for real-time identification of parathyroid glands, as well as aiding in differentiating between normal and abnormal glands. Tables 1,2 provide practical guides for the use of NIRAF devices and contrast-enhanced parathyroid imaging during parathyroidectomy. Studies have demonstrated autofluorescence, either by image-based or probe-based devices, identified 91–100% of parathyroid glands during parathyroidectomy for PHPT (32,43-45). In one series of patients with parathyroid disease and negative preoperative localization, 21% of abnormal glands were recognized with autofluorescence before visual identification by the surgeon (46). Hyperfunctioning parathyroid glands have been shown to exhibit a heterogeneous pattern of autofluorescence and lower average autofluorescence intensity than normal parathyroid glands (Figure 4) (44). Often in parathyroid adenomas, there is a “cap” of normal tissue at one end, which may appear as a rim of intense signal compared to the remainder of the gland on image-based NIRAF (45). A negative correlation between serum calcium and intensity of autofluorescence has been described, as well as between serum PTH and autofluorescence in renal hyperparathyroidism only (43). Interestingly, glands in renal hyperparathyroidism appear to have less fluorescence overall, particularly in glands with diffuse chief cell hyperplasia. Glands with oxyphil predominance tend to have increased autofluorescence (32,43).
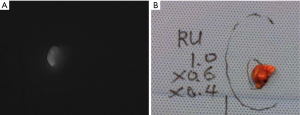
When used for parathyroid localization and assessment of perfusion during parathyroidectomy, ICG fluorescence imaging is typically performed using a double injection protocol; the ICG is injected once the central neck is exposed and the thyroid retracted medially on each side (39). In one study, 93% of identified parathyroid glands demonstrated ICG uptake overall. Using a graded scale, a higher degree of fluorescence was seen in patients with serum calcium levels >11 mg/dL, and in glands larger than 10 mm (41). ICG fluorescence imaging may be particularly useful during subtotal parathyroidectomy in order to evaluate the viability of the parathyroid remnant. Typically, the most normal looking gland, based on visual evaluation of its color and subjective appearance, is left in place or chosen for subtotal resection. ICG fluorescence imaging theoretically decreases the risk of permanent post-operative hypoparathyroidism by confirming that the parathyroid remnant is well-perfused before resecting the other glands. In a small case series utilizing this technique, no patients had permanent hypoparathyroidism, but ICG fluorescence imaging did not prevent immediate post-operative hypocalcemia in this high-risk population (47). In a small study of patients with secondary hyperparathyroidism, patients undergoing ICG fluorescence imaging had shorter operative times, and lower rate of persistent hyperparathyroidism, suggesting possible benefit in this population (48).
Significance and clinical applications
Parathyroid fluorescence, whether probe-based or image-based detection of NIRAF or contrast-enhanced imaging with ICG, has emerged as a useful adjunct for the identification and preservation of parathyroid glands during both thyroidectomy and parathyroidectomy. Tables 1,2 provide practical guidance for the use of these modalities, and for integrating them into surgical practice, while Table 3 highlights some of the distinct advantages and limitations of the various modalities.
Table 3
Image-based NIRAF | Probe-based NIRAF | Contrast-enhanced fluorescence | |
---|---|---|---|
Advantages | Contactless | Real-time quantitative fluorescent intensity measurements | Provides assessment of parathyroid gland perfusion and viability |
Provides wide field of view for gland “mapping” | Audible signal for positive identification | ||
Reusable device | Unaffected by normal operating room lights | ||
Multiuse; may be used for other fluorescent imaging | Compact device can fit in small incisions | ||
Limitations | Operator dependent for use and interpretation | Requires contact with tissue in specific area of interest (typically requiring visual identification prior to device confirmation) | Requires fluorophore injection (with ICG, possible allergic reaction in patients with iodine allergy) |
Optimal view requires ambient lights to be turned off* | Requires re-calibration for each patient | Evaluation by NIRAF not possible after ICG injection | |
Cannot assess gland perfusion or viability without contrast administration | Cannot assess gland perfusion or viability |
*, Newer Fluobeam LX advertised as compatible with ambient lights; data pending. NIRAF, near-infrared autofluorescence; ICG, indocyanine green.
Current surgical training teaches parathyroid identification by anatomic mapping and visual assessment of subtle variations in gross pathology. Years of operative experience and higher operative volume can improve rates of parathyroid identification and preservation during thyroidectomy (49,50), but surgical techniques such NIRAF and contrast-enhanced fluorescence may be particularly beneficial as educational adjuncts for residents or less experienced surgeons in training. These techniques can assist the surgeon in identifying the glands, as well as, in the case of contrast-enhanced angiography, their feeding vessels. Being able to accurately identify parathyroid glands in real-time noninvasively could avoid unnecessary time-consuming, costly, and potentially injurious frozen section biopsy (23).
The cost-effectiveness of these techniques remains uncertain. The current FDA-approved commercial devices require initial costs for hardware, as well as costs per procedure for consumables (sterile sheath for the image-based device and probe for the probe-based device). In addition to the FDA-approved commercial devices discussed above, several lab-built non-commercial devices have also been described, including a Raspberry Pi-based device (51), modified Karl Storz NIR endoscopic system (52-54), and filtered light emitting diode (LED) plus night vision goggle system (55). The goal of these modified systems is to provide a lower-cost method for capturing parathyroid autofluorescence using less expensive, or already available, equipment. With an estimated 82,000 total thyroidectomies and parathyroidectomies performed in the United States every year (56,57), increasing incidence of thyroid cancer and thyroid operations (56,58), and significant healthcare resource utilization of patients with chronic hypoparathyroidism (59), even a small reduction in surgical complications through accurate and efficient parathyroid identification with fluorescent technologies could have a positive economic impact (23). However, an accurate analysis of cost-effectiveness of these techniques will require more robust and long-term data on their clinical benefits (45).
Conclusions
In this chapter, we have described some of the benefits and pitfalls of image-based and probe-based NIRAF and contrast-enhanced fluorescence imaging with ICG, but all have been shown to be accurate in parathyroid identification. The techniques described are safe, require no radiation, and can be easily integrated into the surgical workflow. They may be useful in teaching trainees to find and preserve parathyroid glands. Long-term data on post-thyroidectomy hypoparathyroidism and cure of hyperparathyroidism using these techniques is currently lacking, as is evaluation of their cost-effectiveness, and the results of ongoing appropriately powered trials may elucidate their true clinical impact.
Acknowledgments
Funding: None.
Footnote
Provenance and Peer Review: This article was commissioned by the Guest Editors (Marcin Barczyński and Maurizio Iacobone) for the series “Recent Challenges in the Management of Thyroid Tumors” published in Annals of Thyroid. The article has undergone external peer review.
Conflicts of Interest: Both authors have completed the ICMJE uniform disclosure form (available at http://dx.doi.org/10.21037/aot-20-56). The series “Recent Challenges in the Management of Thyroid Tumors” was commissioned by the editorial office without any funding or sponsorship. The authors have no other conflicts of interest to declare.
Ethical Statement: The authors are accountable for all aspects of the work in ensuring that questions related to the accuracy or integrity of any part of the work are appropriately investigated and resolved.
Open Access Statement: This is an Open Access article distributed in accordance with the Creative Commons Attribution-NonCommercial-NoDerivs 4.0 International License (CC BY-NC-ND 4.0), which permits the non-commercial replication and distribution of the article with the strict proviso that no changes or edits are made and the original work is properly cited (including links to both the formal publication through the relevant DOI and the license). See: https://creativecommons.org/licenses/by-nc-nd/4.0/.
References
- Sonne-Holm E, Holst Hahn C. Prolonged Duration of Surgery Predicts Postoperative Hypoparathyroidism among Patients Undergoing Total Thyroidectomy in a Tertiary Referral Centre. Eur Thyroid J 2017;6:255-62. [Crossref] [PubMed]
- Bergenfelz A, Nordenström E, Almquist M. Morbidity in patients with permanent hypoparathyroidism after total thyroidectomy. Surgery 2020;167:124-8. [Crossref] [PubMed]
- Paras C, Keller M, White L, et al. Mahadevan-Jansen A. Near-infrared autofluorescence for the detection of parathyroid glands. J Biomed Opt 2011;16:067012. [Crossref] [PubMed]
- Tanaka E, Choi HS, Fujii H, et al. Image-guided oncologic surgery using invisible light: completed pre-clinical development for sentinel lymph node mapping. Ann Surg Oncol 2006;13:1671-81. [Crossref] [PubMed]
- McWade MA, Paras C, White LM, et al. Mahadevan-Jansen A, Broome JT. A novel optical approach to intraoperative detection of parathyroid glands. Surgery 2013;154:1371-7; discussion 1377. [Crossref] [PubMed]
- FDA permits marketing of two devices that detect parathyroid tissue in real-time during surgery [Internet]. FDA, 2020 [cited 2020 Aug 5]. Available from: https://www.fda.gov/news-events/press-announcements/fda-permits-marketing-two-devices-detect-parathyroid-tissue-real-time-during-surgery
- De Leeuw F, Breuskin I, Abbaci M, et al. Intraoperative Near-infrared Imaging for Parathyroid Gland Identification by Auto-fluorescence: A Feasibility Study. World J Surg 2016;40:2131-8. [Crossref] [PubMed]
- Demarchi MS, Karenovics W, Bédat B, et al. Intraoperative Autofluorescence and Indocyanine Green Angiography for the Detection and Preservation of Parathyroid Glands. J Clin Med 2020;9:830. [Crossref] [PubMed]
- Orloff LA, Wiseman SM, Bernet VJ, et al. American Thyroid Association Statement on Postoperative Hypoparathyroidism: Diagnosis, Prevention, and Management in Adults. Thyroid 2018;28:830-41. [Crossref] [PubMed]
- Edafe O, Antakia R, Laskar N, et al. Systematic review and meta-analysis of predictors of post-thyroidectomy hypocalcaemia. Br J Surg 2014;101:307-20. [Crossref] [PubMed]
- Almquist M, Ivarsson K, Nordenström E, et al. Mortality in patients with permanent hypoparathyroidism after total thyroidectomy. Br J Surg 2018;105:1313-8. [Crossref] [PubMed]
- Gourgiotis S, Moustafellos P, Dimopoulos N, et al. Inadvertent parathyroidectomy during thyroid surgery: the incidence of a complication of thyroidectomy. Langenbecks Arch Surg 2006;391:557-60. [Crossref] [PubMed]
- Rix TE, Sinha P. Inadvertent parathyroid excision during thyroid surgery. Surgeon 2006;4:339-42. [Crossref] [PubMed]
- Khairy GA, Al-Saif A. Incidental parathyroidectomy during thyroid resection: incidence, risk factors, and outcome. Ann Saudi Med 2011;31:274-8. [Crossref] [PubMed]
- Zhou HY, He JC, McHenry CR. Inadvertent parathyroidectomy: incidence, risk factors, and outcomes. J Surg Res 2016;205:70-5. [Crossref] [PubMed]
- Olson JA, DeBenedetti MK, Baumann DS, et al. Parathyroid autotransplantation during thyroidectomy. Results of long-term follow-up. Ann Surg 1996;223:472-8; discussion 478-80. [Crossref] [PubMed]
- McLeod IK, Arciero C, Noordzij JP, et al. The use of rapid parathyroid hormone assay in predicting postoperative hypocalcemia after total or completion thyroidectomy. Thyroid 2006;16:259-65. [Crossref] [PubMed]
- Falco J, Dip F, Quadri P, et al. Increased identification of parathyroid glands using near infrared light during thyroid and parathyroid surgery. Surg Endosc 2017;31:3737-42. [Crossref] [PubMed]
- Abbaci M, De Leeuw F, Breuskin I, et al. Parathyroid gland management using optical technologies during thyroidectomy or parathyroidectomy: A systematic review. Oral Oncol 2018;87:186-96. [Crossref] [PubMed]
- Moore EC, Rudin A, Alameh A, et al. Near-infrared imaging in re-operative parathyroid surgery: first description of autofluorescence from cryopreserved parathyroid glands. Gland Surg 2019;8:283-6. [Crossref] [PubMed]
- Kahramangil B, Dip F, Benmiloud F, et al. Detection of Parathyroid Autofluorescence Using Near-Infrared Imaging: A Multicenter Analysis of Concordance Between Different Surgeons. Ann Surg Oncol 2018;25:957-62. [Crossref] [PubMed]
- Falco J, Dip F, Quadri P, et al. Cutting Edge in Thyroid Surgery: Autofluorescence of Parathyroid Glands. J Am Coll Surg 2016;223:374-80. [Crossref] [PubMed]
- Solórzano CC, Thomas G, Baregamian N, et al. Detecting the Near Infrared Autofluorescence of the Human Parathyroid: Hype or Opportunity? Ann Surg 2020;272:973-85. [Crossref] [PubMed]
- Benmiloud F, Rebaudet S, Varoquaux A, et al. Impact of autofluorescence-based identification of parathyroids during total thyroidectomy on postoperative hypocalcemia: a before and after controlled study. Surgery 2018;163:23-30. [Crossref] [PubMed]
- Dip F, Falco J, Verna S, et al. Randomized Controlled Trial Comparing White Light with Near-Infrared Autofluorescence for Parathyroid Gland Identification During Total Thyroidectomy. J Am Coll Surg 2019;228:744-51. [Crossref] [PubMed]
- Benmiloud F, Godiris-Petit G, Gras R, et al. Association of Autofluorescence-Based Detection of the Parathyroid Glands During Total Thyroidectomy With Postoperative Hypocalcemia Risk: Results of the PARAFLUO Multicenter Randomized Clinical Trial. JAMA Surg 2020;155:106-12. [Crossref] [PubMed]
- Kim YS, Erten O, Kahramangil B, et al. The impact of near infrared fluorescence imaging on parathyroid function after total thyroidectomy. J Surg Oncol 2020;122:973-9. [PubMed]
- DiMarco A, Chotalia R, Bloxham R, et al. Does fluoroscopy prevent inadvertent parathyroidectomy in thyroid surgery? Ann R Coll Surg Engl 2019;101:508-13. [Crossref] [PubMed]
- Thomas G, McWade MA, Paras C, et al. Developing a Clinical Prototype to Guide Surgeons for Intraoperative Label-Free Identification of Parathyroid Glands in Real Time. Thyroid 2018;28:1517-31. [Crossref] [PubMed]
- Solórzano CC, Thomas G, Berber E, et al. Current state of intraoperative use of near infrared fluorescence for parathyroid identification and preservation. Surgery 2020; Epub ahead of print. [Crossref] [PubMed]
- Thomas G, Squires MH, Metcalf T, et al. Imaging or Fiber Probe-Based Approach? Assessing Different Methods to Detect Near Infrared Autofluorescence for Intraoperative Parathyroid Identification. J Am Coll Surg 2019;229:596-608.e3. [Crossref] [PubMed]
- McWade MA, Sanders ME, Broome JT, et al. Establishing the clinical utility of autofluorescence spectroscopy for parathyroid detection. Surgery 2016;159:193-202. [Crossref] [PubMed]
- Alander JT, Kaartinen I, Laakso A, et al. A review of indocyanine green fluorescent imaging in surgery. Int J Biomed Imaging 2012;2012:940585. [Crossref] [PubMed]
- Vidal Fortuny J, Belfontali V, Sadowski SM, et al. Parathyroid gland angiography with indocyanine green fluorescence to predict parathyroid function after thyroid surgery. Br J Surg 2016;103:537-43. [Crossref] [PubMed]
- Graves C, Ely S, Idowu O, et al. Direct Gallbladder Indocyanine Green Injection Fluorescence Cholangiography During Laparoscopic Cholecystectomy. J Laparoendosc Adv Surg Tech A 2017;27:1069-73. [Crossref] [PubMed]
- Lang BH, Wong CK, Hung HT, et al. Indocyanine green fluorescence angiography for quantitative evaluation of in situ parathyroid gland perfusion and function after total thyroidectomy. Surgery 2017;161:87-95. [Crossref] [PubMed]
- Vidal Fortuny J, Sadowski SM, Belfontali V, et al. Randomized clinical trial of intraoperative parathyroid gland angiography with indocyanine green fluorescence predicting parathyroid function after thyroid surgery. Br J Surg 2018;105:350-7. [Crossref] [PubMed]
- Razavi AC, Ibraheem K, Haddad A, et al. Efficacy of indocyanine green fluorescence in predicting parathyroid vascularization during thyroid surgery. Head Neck 2019;41:3276-81. [Crossref] [PubMed]
- Spartalis E, Ntokos G, Georgiou K, et al. Intraoperative Indocyanine Green (ICG) Angiography for the Identification of the Parathyroid Glands: Current Evidence and Future Perspectives. In Vivo 2020;34:23-32. [Crossref] [PubMed]
- Rudin AV, McKenzie TJ, Thompson GB, et al. Evaluation of Parathyroid Glands with Indocyanine Green Fluorescence Angiography After Thyroidectomy. World J Surg 2019;43:1538-43. [Crossref] [PubMed]
- Zaidi N, Bucak E, Okoh A, et al. The utility of indocyanine green near infrared fluorescent imaging in the identification of parathyroid glands during surgery for primary hyperparathyroidism. J Surg Oncol 2016;113:771-4. [Crossref] [PubMed]
- Walker MD, Silverberg SJ. Primary hyperparathyroidism. Nat Rev Endocrinol 2018;14:115-25. [Crossref] [PubMed]
- DiMarco A, Chotalia R, Bloxham R, et al. Autofluorescence in Parathyroidectomy: Signal Intensity Correlates with Serum Calcium and Parathyroid Hormone but Routine Clinical Use is Not Justified. World J Surg 2019;43:1532-7. [Crossref] [PubMed]
- Kose E, Kahramangil B, Aydin H, et al. Heterogeneous and low-intensity parathyroid autofluorescence: Patterns suggesting hyperfunction at parathyroid exploration. Surgery 2019;165:431-7. [Crossref] [PubMed]
- Di Marco AN, Palazzo FF. Near-infrared autofluorescence in thyroid and parathyroid surgery. Gland Surg 2020;9:S136-46. [Crossref] [PubMed]
- Kose E, Rudin AV, Kahramangil B, et al. Autofluorescence imaging of parathyroid glands: An assessment of potential indications. Surgery 2020;167:173-9. [Crossref] [PubMed]
- Vidal Fortuny J, Sadowski SM, Belfontali V, et al. Indocyanine Green Angiography in Subtotal Parathyroidectomy: Technique for the Function of the Parathyroid Remnant. J Am Coll Surg 2016;223:e43-9. [Crossref] [PubMed]
- Cui L, Gao Y, Yu H, et al. Intraoperative Parathyroid Localization with Near-Infrared Fluorescence Imaging Using Indocyanine Green during Total Parathyroidectomy for Secondary Hyperparathyroidism. Sci Rep 2017;7:8193. [Crossref] [PubMed]
- Attie JN, Khafif RA. Preservation of parathyroid glands during total thyroidectomy: Improved technic utilizing microsurgery. Am J Surg 1975;130:399-404. [Crossref] [PubMed]
- Meltzer C, Hull M, Sundang A, et al. Association Between Annual Surgeon Total Thyroidectomy Volume and Transient and Permanent Complications. JAMA Otolaryngol Head Neck Surg 2019; Epub ahead of print. [Crossref] [PubMed]
- Kim Y, Kim SW, Lee KD, et al. Real-time localization of the parathyroid gland in surgical field using Raspberry Pi during thyroidectomy: a preliminary report. Biomed Opt Express 2018;9:3391-8. [Crossref] [PubMed]
- Ladurner R, Sommerey S, Arabi NA, et al. Intraoperative near-infrared autofluorescence imaging of parathyroid glands. Surg Endosc 2017;31:3140-5. [Crossref] [PubMed]
- Wolf HW, Grumbeck B, Runkel N. Intraoperative verification of parathyroid glands in primary and secondary hyperparathyroidism using near-infrared autofluorescence (IOPA). Updates Surg 2019;71:579-85. [Crossref] [PubMed]
- Ladurner R, Lerchenberger M, Al Arabi N, et al. Parathyroid Autofluorescence-How Does It Affect Parathyroid and Thyroid Surgery? A 5 Year Experience. Molecules 2019;24:2560. [Crossref] [PubMed]
- Serra C, Silveira L, Canudo A, et al. Parathyroid identification by autofluorescence - preliminary report on five cases of surgery for primary hyperparathyroidism. BMC Surg 2019;19:120. [Crossref] [PubMed]
- Sosa JA, Hanna JW, Robinson KA, et al. Increases in thyroid nodule fine-needle aspirations, operations, and diagnoses of thyroid cancer in the United States. Surgery 2013;154:1420-6; discussion 1426-7. [Crossref] [PubMed]
- Kim SM, Shu AD, Long J, et al. Declining Rates of Inpatient Parathyroidectomy for Primary Hyperparathyroidism in the US. PloS One 2016;11:e0161192. [Crossref] [PubMed]
- Lim H, Devesa SS, Sosa JA, et al. Trends in Thyroid Cancer Incidence and Mortality in the United States, 1974-2013. JAMA 2017;317:1338-48. [Crossref] [PubMed]
- Chen K, Krasner A, Li N, et al. Clinical burden and healthcare resource utilization among patients with chronic hypoparathyroidism, overall and by adequately vs not adequately controlled disease: a multi-country chart review. J Med Econ 2019;22:1141-52. [Crossref] [PubMed]
Cite this article as: Graves CE, Duh QY. Fluorescent technologies for intraoperative parathyroid identification. Ann Thyroid 2020;5:26.